Deciphering Gene Expression in Industrially Relevant Bacterial Isolates: Molecular Insights and Applications
Gene expression studies in industrial bacterial isolates are crucial for optimizing their metabolic potential in biotechnology. This blog explores key steps, including strain selection, growth optimization, RNA isolation, cDNA synthesis, and PCR-based analysis, highlighting their role in enzyme production, bioremediation, and biofuel development.
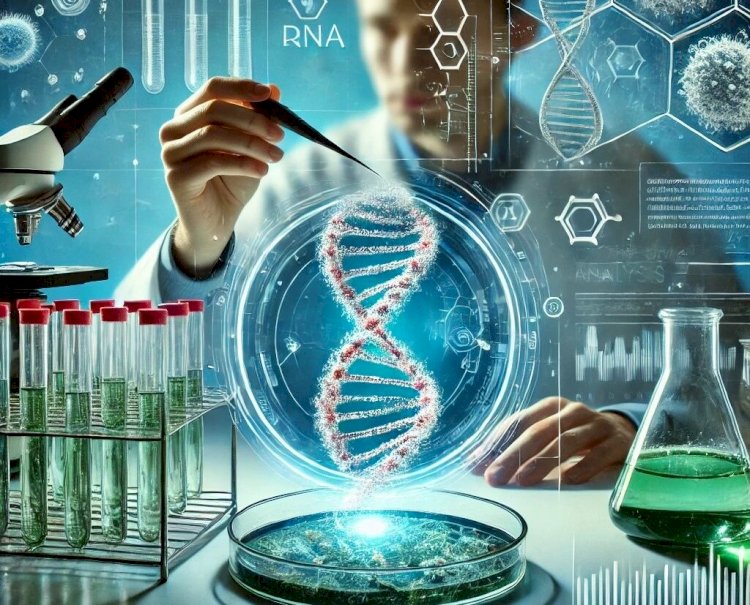
Deciphering Gene Expression in Industrially Relevant Bacterial Isolates: Molecular Insights and Applications
In order to comprehend the molecular mechanisms underlying bacterial metabolism, control, and industrial applications, gene expression investigations are essential. The capacity of industrially significant bacterial strains to create bioactive chemicals, metabolites, and enzymes is the subject of much research. This blog discusses the methods for analyzing gene expression in these bacterial isolates, including RNA isolation, strain selection, growth characterization, and gene amplification.
- Selection of Industrially Important Bacterial Strains:
Choosing a bacterial strain having industrial relevance is the initial stage in gene expression research. These bacteria are selected because they can create bioactive substances, helpful enzymes (including lipases, amylases, and proteases), or because they play a part in fermentation and bioremediation. Streptomyces species, Escherichia coli, and Bacillus subtilis are typical examples.
- Growth Parameters and Characteristics:
To maximize growth and product generation, the chosen bacterial strain is grown in a controlled environment. Important parameters consist of:
- Temperature: The ideal temperature for growth (for example, 37°C is ideal for B. subtilis development).
- pH: Preserving the optimal pH for the stability and activity of enzymes.
- The selection of carbon and nitrogen sources to improve gene expression is known as nutrient composition.
- Providing aerobic bacteria with a sufficient supply of oxygen is achieved by aeration and agitation.
- Identification and Characterization:
The right strain of bacteria is chosen for industrial purposes when isolates are accurately identified and described. This procedure entails:
- Morphological Analysis: Observing colony structure, Gram staining, and motility characteristics.
- Biochemical Characterization: Assessing metabolic capacities by performing tests including glucose fermentation, urease generation, oxidase, and catalase.
- Analyzing the production of enzymes and biochemical processes necessary for commercial uses, such as cellulase, amylase, and protease assays, is known as metabolic profiling.
- Total RNA Isolation and Quantification:
To investigate gene expression, RNA isolation is carried out after the bacterial strain has been described. The procedure consists of:
- Cell lysis: Breaking down bacterial cell walls to liberate RNA by chemical (phenol-chloroform), mechanical (bead beating), or enzymatic (lysozyme treatment) means.
- RNA stabilization is the process of maintaining RNA integrity by stopping degradation with RNase inhibitors or by instantly freezing it in liquid nitrogen.
- Purification is the process of removing proteins, DNA, and other impurities from high-quality RNA by employing spin-column (silica-based) or TRIzol-based procedures.
- Quantification and Evaluation of Integrity: Measuring RNA concentration using spectrophotometric (NanoDrop) or fluorometric (Qubit) methods to determine RNA yield and purity, ensuring accurate quantification for downstream applications. Verifying RNA integrity by performing denaturing gel electrophoresis or using an Agilent Bioanalyzer to assess ribosomal RNA banding patterns, identifying potential degradation or contamination. Removing genomic DNA contamination by treating RNA samples with DNase-I, preventing interference in downstream processes such as reverse transcription, quantitative PCR, or RNA sequencing.
- RNA to cDNA Conversion Using Reverse Transcription (RT-PCR):
To study gene expression, total RNA is first converted into complementary DNA (cDNA) through reverse transcription. This process includes:
- Primer Selection: Utilizing random hexamers for broad cDNA synthesis or gene-specific primers for targeted transcription.
- Reverse Transcriptase Enzyme: Using high-fidelity enzymes like M-MLV (Moloney Murine Leukemia Virus) or SuperScript III to ensure efficient cDNA generation.
- Reaction Optimization:
- Conducting reverse transcription at optimal temperatures (42–50°C for 30–60 minutes) to achieve complete cDNA synthesis.
- Incorporating necessary components such as reaction buffers, dNTPs, and DTT to maintain enzyme stability.
- Running a negative control (lacking reverse transcriptase) to identify potential genomic DNA contamination.
- Quality Assessment: Evaluating cDNA integrity through agarose gel electrophoresis or pre-amplification quality control using qPCR with housekeeping genes.
- Gene Amplification Using cDNA as a Template:
Again, Polymerase Chain Reaction (PCR) is used to amplify target genes from the synthesized cDNA. This process consists of:
- Primer Design: Designing gene-specific primers using bioinformatics tools such as Primer-BLAST, ensuring high specificity and efficiency.
- PCR Optimization:
- Adjusting reaction conditions such as annealing temperature, magnesium ion concentration, and cycle number for optimal amplification.
- Using high-fidelity DNA polymerases like Q5 or Phusion for accurate amplification.
- Running negative controls (without cDNA template) to rule out contamination.
- Amplification Process: Performing denaturation (95°C), annealing (optimized temperature), and extension (72°C) cycles using a thermal cycler. Conducting a gradient PCR to determine the best annealing temperature for specific primers.
- Validation:
- Running amplified products on a 1–2% agarose gel stained with ethidium bromide or SYBR Safe.
- Comparing amplicon sizes to a DNA ladder for expected fragment length.
- If needed, cloning PCR products into plasmid vectors for sequencing validation.
- Gene Expression Using Semi-Quantitative PCR:
Semi-quantitative PCR (sqPCR) allows for the comparative analysis of gene expression under different conditions. Housekeeping genes such as 16S rRNA, serve as internal controls to normalize expression levels. The process involves:
- PCR Setup:
- Running reactions in triplicates to improve data accuracy and reproducibility.
- Using optimized reaction conditions for specific gene amplification.
- Visualization:
- Loading PCR products onto a 1.5% agarose gel stained with intercalating dyes such as ethidium bromide.
- Running electrophoresis at a constant voltage (80–100V) for proper DNA separation.
- Quantification:
- Capturing gel images under UV transillumination and analyzing band intensities using densitometry software such as ImageJ.
- Normalizing expression levels relative to housekeeping genes for reliable comparisons.
- Alternative Quantitative Approach: For precise gene expression analysis, real-time PCR (qPCR) is performed using SYBR Green or TaqMan probes to quantify transcript levels dynamically.
- Role of IBRI Noida in Hands-on Training:
The Indian Biological Sciences and Research Institute (IBRI), Noida, plays a significant role in providing hands-on training in molecular biology techniques for gene expression studies. IBRI offers structured workshops and training programs that equip researchers and students with practical knowledge in:
- Bacterial Identification and Characterization: Training in morphological, biochemical, and molecular techniques to accurately identify industrially relevant bacterial strains.
- RNA Extraction and Quality Assessment: Hands-on experience in isolating high-quality RNA from bacterial cultures and assessing integrity using spectrophotometric and electrophoretic methods.
- cDNA Synthesis and PCR Optimization: Practical exposure to reverse transcription, primer design, and polymerase chain reaction techniques to amplify target genes.
- Gene Expression Analysis: Training in semi-quantitative and real-time PCR methods for analyzing bacterial gene expression under different environmental and industrial conditions.
- Bioinformatics and Data Analysis: Guidance on using computational tools for sequence analysis, primer design, and interpretation of gene expression data.
Through these specialized programs, IBRI Noida bridges the gap between theoretical knowledge and industrial application, preparing researchers for careers in biotechnology, microbiology, and molecular genetics.
Conclusion:
Investigating gene expression in industrially significant bacterial isolates provides valuable insights into microbial metabolism and their potential in biotechnology. By employing a structured methodology, from selecting bacterial strains to gene amplification and expression analysis, researchers can enhance strain performance for improved productivity. Such studies play a crucial role in developing genetically optimized strains for applications in pharmaceutical production, bioremediation, enzyme manufacturing, and biofuel synthesis, ultimately driving progress in sustainable industrial biotechnology.
Explore IBRI’s programs today to learn more about molecular biology trainings and unlock new career opportunities and stay ahead in the life sciences industry.
You can contact us at - Email: info@ibri.org.in or call at: (+91) 9999509892, 9971441910